After the renowned theory of stimulated emission was raised by Einstein, the invention of the laser (Light Amplification by Stimulated Emission of Radiation) in 1960 ushered in a new era of light-based technologies. Today, lasers are indispensable in communication, precision manufacturing, and scientific research, etc. But the direct observation of how stimulated emission initiates and evolves inside a laser system, even over a half-century after its invention, has remained a crucial missing piece in this fundamental process.
Now, scientists from the Chinese Academy of Sciences' Shanghai institute of optics and fine mechanics (SIOM) have unraveled this mystery using a novel form of light – electromagnetic waves with massive electrons that float along the surface of a waveguide surface, dubbed also surface plasmon polariton (SPP). The study, published in a recent edition of Nature on Noverber 2, 2022, was led by Prof. Ruxin Li and Prof. TIAN Ye of SIOM and resulted in a powerful, yet ultra-small coherent surface light source that could fit on a microchip.

Fig. 1: Spatiotemporal dynamics of the electromagnetic field of the SPPs: Each panel is a recorded frame of the magnetic field of the SPPs projected at a magneto-optical crystal and at different time delays. Credit: Zhang et al Nature (2022). (Image by SIOM)
As a comparatively new light form, SPP is gaining more attention over the past decades due to its distinctive characteristics of sub-wavelength confinement and near-field enhancement. A wide scope of interesting applications is hence opened utilizing the SPPs, such as integrated photonic circuits and highly sensitive detectors. Yet one major obstacle towards these applications is the relatively low coupling efficiency that restricts the SPPs to a tiny spatial size and low energy.
To coherently amplify the SPP, just like a laser, an innovative new way is employed in the research which takes advantage of free electrons generated by a femtosecond laser pulse. The free electrons coprapagates with the SPP over the waveguide surface in a synchronous manner, and transfer their energy to the SPP via the stimulated emission. Consequently, the spatial-temporal evolution of the SPP-electron interaction is captured by an ultrafast probe light that records the electric and magnetic field variances of the SPP with femtosecond resolution.
"This way of making laser-like light could surpass existing methods that use electrons," writing in the News & Views of Nature that comments on the work by Dr. Nicholas Rivera, a scientist at Harvard University, who was not connected with this work. "The effects reported here could be amplified and enhanced by structures whose electromagnetic properties vary in space (for example, artificial materials called photonic crystals or metamaterials). Such spatially structured materials can offer marked control over the behaviour of light - a capability that has been used extensively in the field of nanophotonics to shape the spontaneous and stimulated emission of visible and infrared light."
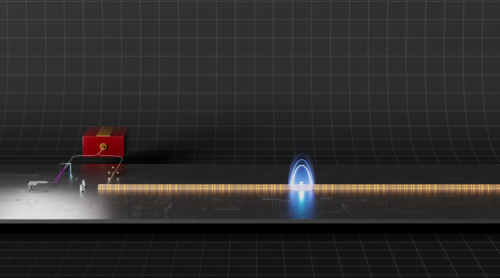
Fig. 2: Concept of a future on-chip SPP laser: Electrons are generated through photoemission from a nanotip mounted upon the chip. A fiber laser system provides the excitation laser pulse for triggering the electrons as well as the weak SPP seed that travels on a waveguide embedded in the chip. For phase-matched conditions, the electrons intensify the SPP in a copropagating manner so as to facilitate continuous SPP amplification. At the end of the waveguide, the intensified SPP can be disseminated to multiple applications which can also be integrated on the scale of a miniature chip. Credit: Kaiquan Jiang. (Image by SIOM)
After showing the stimulated emission process, the research group then worked to turn their observed phenomena into a miniature laser. They did so by adding nanotip-based electron sources to the waveguide surface that could provide phase-matched electrons with SPP. Simulations suggest the SPP power could become even stronger when a proper electron pulse is used. The predicted power could even be comparable to much larger photonic lasers. Such a high-power coherent SPP light source will allow applications that require power compensation either as stand-alone components or as 'gain blocks' atop a photonic chip. To realize such perspectives, the research group will continue to improve the peak power of the demonstrated coherent SPP light source, and to make it easily integrated into a photonic chip for the diverse applications therein.
Article Website:
https://doi.org/10.1038/s41586-022-05239-2
Contact:
WU Xiufeng
General Administrative Office
Shanghai Institute of Optics and Fine Mechanics, CAS
Email: xfwu@siom.ac.cn
Web: http://english.siom.cas.cn/